In the Nevada desert near Las Vegas, more than 10,000 mirrors focus the sun’s energy on a 640-foot tower. This concentrating solar power, or CSP, plant can generate 110 megawatts of electricity (enough for roughly 18,000 homes) by using the sun’s energy to heat a salt solution. That solution boils water, producing steam that turns a turbine generator. But to run at night or on a cloudy day, the heat-transfer medium – molten salt in this example – must stay hot as long as possible, and that’s a challenge.
“How long you can maintain a high temperature depends on the heat-transfer medium, the insulation of the thermal energy storage tank and so on,” says Dominic Lee, manager in the sustainable electricity program at the Department of Energy’s (DOE) Oak Ridge National Laboratory. A CSP plant’s efficiency also depends on getting as much of the sun’s energy to the heat-transfer medium as possible. (See sidebar, “Maximizing the mirrors.”)
Past CSP plants used organic oils for heat transfer, but molten salt allows a higher temperature. “The molten salt also has a lower vapor pressure,” Lee says, “so you don’t need pressure-rated tanks.”
What’s really needed, he says, is a material that’s exceptionally stable at extreme temperatures, has scant vapor pressure and has a good heat capacity – “something that doesn’t exist or hasn’t been identified.”
‘Even ideal particles are challenging, and we need good agreement between the theoretical and experimental approaches.’
Christine Hrenya, a University of Colorado, Boulder, chemical and biological engineering professor, is trying to find a better medium, with the help of postdoctoral researcher Aaron Morris, Zhiwen Ma of DOE’s National Renewable Energy Laboratory in Golden, Colorado, and Sreekanth Pannala, then at Oak Ridge and now a research fellow at SABIC in Houston. The team, part of the DOE Solar Energy Technologies Office’s SunShot Initiative, has received 20 million processor hours at the Oak Ridge Leadership Computing Facility through the Office of Science’s ASCR (Advanced Scientific Research Computing) Leadership Computing Challenge.
To get the most from CSP, the heat-transfer medium must remain stable above 600 degrees Celsius, about 50 degrees beyond when molten salt loses stability. “With molten salt, you can’t take advantage of all of the sun’s energy in the power tower because the heat-transfer medium becomes a bottleneck in the effectiveness,” Hrenya explains. She wanted something stable over 600 degrees that would also provide good thermal storage. “If you go on the beach long after the sun goes down,” Hrenya says, “the sand is still warm.” So her team decided to try sand-like particles as the medium.
A bucket-conveyor system would take the particles up to the power tower where they’d be heated. Then the particles would fall through a series of tubes, transferring heat to power a steam-driven turbine. “It’s like the Plinko game on ‘The Price is Right,’” Hrenya says, “where people put in a flat disk that slides down a surface and bounces off pegs.” In her CSP system, the particles replace the disk and tubes replace the pegs. After transferring the heat, the conveyor returns the particles to the tower.
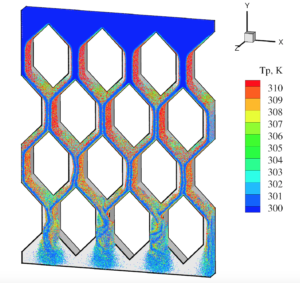
A discrete-particle model created this temperature profile as sand-like particles transfer heat to fluid in a system of tubes. Image courtesy of Christine M. Hrenya, University of Colorado, Boulder.
“With gases or liquids, heat transfer is well known,” Hrenya says, “but we are studying a multiphase system: air plus the sand-like particles.” Her team needed to develop new models.
Using Oak Ridge’s Titan supercomputer, the scientists started with a discrete particle–tracking model. It follows how the grains – up to 13 million of them – move and transfer heat. “That sounds like a lot of particles, but it’s not even close to what we’d find in the system.”
To accommodate a larger system with more particles, the scientists also developed a continuum model. “Here we solve the energy balance and momentum for the whole phase instead of individual particles,” Hrenya says.
Then the team compared results and found the continuum-based model was excellent, Hrenya says. The heat-transfer results differed by less than 15 percent.
The next step is to test the system experimentally. With National Science Foundation backing, Hrenya is starting with ideal data, generated from controlled particles of identical size and shape. “Real particles can be different sizes and have different roughness,” she says, “but even ideal particles are challenging at first, and we need good agreement between the theoretical and experimental approaches.”
Over time, Hrenya will create increasingly realistic systems to test. She will move from motionless particles to moving ones, add random roughness and so on. At each step, she will compare and refine her models so she can ultimately design the most effective heat-transfer medium for a CSP plant – all while she learns more about the fundamental physics that underlies the process.
“If we want to do renewables and do them right, it will take more than one type,” Hrenya says. “Concentrating solar power is a necessary piece, but not the only one.” The more efficient CSP becomes, however, the more useful it will be.