Susan Kurien believes small things can make a big difference.
Kurien, a researcher at the Department of Energy’s Los Alamos National Laboratory, studies how the physics of small-scale ocean phenomena influence large-scale climate. The work she and her fellow researchers do could improve the models scientists rely upon to predict global climate change.
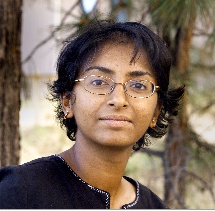
Susan Kurien, Los Alamos National Lab
Small-scale effects are “happening continuously, so eventually you would have to feel them in the large scales,” such as slow-moving currents and long-term climate, says Kurien, who came to Los Alamos as a postdoctoral researcher in 2002. They’re a bit like drops of rain, she says: Each alone is a tiny amount of water, but added together they can cause a massive flood.
One example, Kurien says, is the effect of “dense water overflows” –relatively fast, deep ocean rivers a few hundred meters across. These continuously feed into the global ocean circulation current, a slow, thousand-year flow extending across the world’s oceans over tens of thousands of kilometers.
She adds: “We want to understand the fundamental physics of small-scale fast processes that affect the large, slow processes over long time scales” so computer models can incorporate them.
Of course, when the ocean is the subject, “small” is relative.
“For example, the Pacific Ocean is more than 10,000 kilometers at its widest, so 10 kilometers is “tiny” by comparison, Kurien says, but “it turns out that those scales and the scales smaller than that actually have strong effects on ocean dynamics” over centuries.
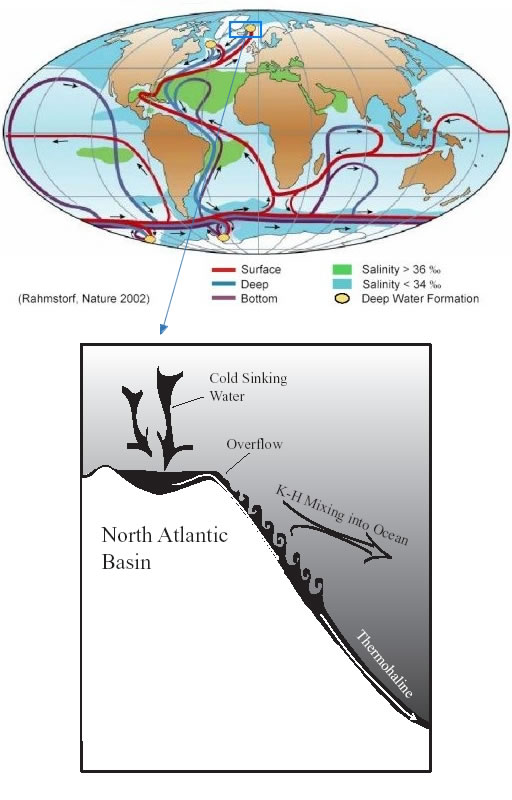
The global ocean circulation current (top) is thousands of miles big and has a thousand-year cycle, but it’s driven by a small, fast phenomenon: The continuous overflow of dense cold water in the deep ocean down to the ocean floor.
It’s often assumed that phenomena smaller than 10 kilometers are irrelevant in ocean dynamics, Kurien says. At around that specific length, called the Rossby deformation radius, two important factors in ocean movement – deformation caused by swirling due to the earth’s rotation, and the propensity of gravity to flatten the ocean – tend to balance each other.
At longer distances, the effect of the earth’s rotation tends to dominate the effect of gravity. Today’s best-resolved ocean models can just about capture dynamics up to the Rossby deformation radius, but no smaller.
Kurien and fellow researchers Beth Wingate of Los Alamos and Leslie Smith and Zhengyu Liu of the University of Wisconsin at Madison believe models must span multiple scales to include the effects of even smaller phenomena.
‘If you don’t put the right equations and physics in, you’re not going to take advantage of what this additional computing ability can give you.’
There’s evidence to support them, now that some computers are powerful enough to simulate ocean activity at smaller scales. A prime example is the Gulf Stream, which pulls warm water from the Gulf of Mexico north to the west coast of Europe. The current has a huge impact on climate.
Models have had difficulty capturing just where and how the Gulf Stream divides as it passes the U.S. Atlantic coast, Kurien says. Capturing that separation is crucial to accurately modeling climate over centuries-long time scales.
When scientists’ models became detailed enough to resolve the Rossby deformation radius, however, all these eddies, all this activity appeared, Kurien says, and the stream separated at the correct location.
The Rossby deformation radius is large enough near the equator to be captured by a model resolving activity on a scale of 10 kilometers. But the radius shrinks at higher latitudes, and is barely captured by the best current models as the Gulf Stream moves north.
“It starts to gain errors as you get to the higher latitudes,” Kurien says. “So the next step is to look at even smaller scales. It doesn’t seem intuitive that the small scales should matter this much, but they do, in the long term.”
Resolving small-scale activity will require computers more powerful than those available today. When they come on line, Kurien wants the computers to run models that incorporate the physics she studies.
“You can throw all the big computers on this problem that you want, but if you don’t put the right equations and physics in, you’re not going to take advantage of what this additional computing ability can give you,” she adds.
Kurien got into the physics of climate after studying turbulence for her doctoral dissertation at Yale University. Since becoming a full-time Los Alamos staff member in 2004, she’s gotten a better idea of the impact her research can have. That’s a change from graduate work, which focuses heavily on a specific subject or problem.
Kurien may not personally insert her group’s characterization of small-scale ocean phenomena into climate models. Yet, she hopes to work with those who do design and code the large models.
“I know that this new physics has to be in the models,” she adds. “And the person who puts it into the model doesn’t necessarily research the physics of it, and so somebody has to work on the physics piece.”
Understanding how her work fits into the larger mission of DOE and climate research is one of the things Kurien likes about working in a national laboratory. The India native thought she would work in academia, but her postdoctoral experience – and exposure to New Mexico’s natural beauty – changed her mind.
“I really liked the people I was working with,” she says, adding “I was given a lot of freedom to pursue my interests, and had very good mentoring.”