Ravi Samtaney’s work is a study in extremes.
His computer codes simulate what happens when pellets of hydrogen isotopes frozen to near absolute zero are shot into a plasma cloud more than six times hotter than the sun’s interior.
The hydrogen pellets are smaller than pencil erasers. The chamber they enter may be two or three stories tall.
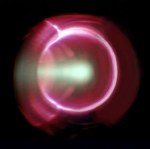
Plasma image following injection of a frozen deuterium pellet. Credit: TFTR Project, PPPL
The pellets vaporize in milliseconds; yet Samtaney, a researcher at the Department of Energy’s Princeton Plasma Physics Laboratory, must break the process into hundreds of steps.
The simulation is important for nuclear fusion – the process behind the sun’s power and a possible source of abundant, clean energy on earth.
A fusion reactor that produces more energy than it consumes is the goal of ITER, a collaboration between the United States and several other countries. ITER, to be built in France, will house a five-story-tall torus, or donut-shaped chamber, called a tokamak.
Powerful magnetic fields will hold the fiery plasma in the torus. The high temperature will strip electrons from the hydrogen atoms and the resulting charged particles will spin through the circular cavity, fusing and releasing energy.
Samtaney’s codes simulate refueling – the injection of frozen hydrogen isotope pellets to keep the reaction going. (Different isotopes of an element have the same number of protons, but different numbers of neutrons, in their nucleus.)
The simulation must accurately depict what happens to the tiny pellet as it’s swallowed by a plasma cloud at least 1,000 times its size – and do it so the program runs in days, rather than years, on some of the world’s fastest computers.
Like many computer simulations, Samtaney’s code to model fuel pellet injection in a fusion reactor uses data meshes. Meshes distribute data points throughout the domain being modeled, then calculate what’s happening at each point. Taken together, those points simulate a continuous process.
The method allowed the refueling simulation to run hundreds of times faster than it would have with a uniform mesh.
But as the size of the reactor being modeled grows, a mesh that distributes points uniformly becomes too large to compute efficiently. If the pellet has a 1-millimeter radius and the tokamak has a 1-meter radius at its narrowest point, “then that’s three orders of magnitude in linear dimensions” that must be modeled, Samtaney says. That and other factors make computing using a uniform grid hugely demanding.
Samtaney turned to Adaptive Mesh Refinement (AMR) to surmount the challenge. AMR conserves computer resources by focusing data points in areas of the most interest – around the pellet – and putting fewer points elsewhere.
Samtaney worked with the Advanced Numerical Algorithms Group at the Department of Energy’s Lawrence Berkeley National Laboratory (LBNL) to add AMR to his physics codes. DOE’s Scientific Discovery through Advanced Computing (SciDAC) program supported the work.
The method allowed the refueling simulation to run hundreds of times faster than it would have with a uniform mesh. It found that where the pellet ends up largely depends on where it starts.
Samtaney’s fusion refueling simulation ran on computer clusters at Princeton and on supercomputers at the National Energy Research Scientific Computing Center at the Berkeley lab.
As the frozen hydrogen isotope pellet enters the plasma, its outer layers ablate, turning to gas. A pocket of high-pressure gas forms and the pellet moves across the magnetic lines of force containing the plasma.
Samtaney’s simulation aligned with experimental findings that show launching pellets from outside the torus – where the magnetic field is relatively weak – makes them stall, so the fuel disperses at the plasma edge. Pellets injected from inside the torus – where the magnetic field is strongest – end up at the center of the plasma, right where they’re wanted.
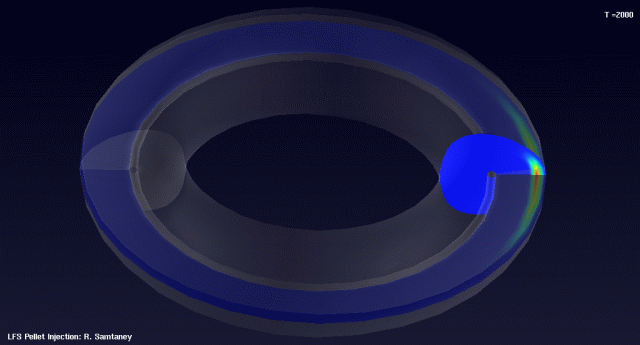
This visualization of the reactor fueling simulation shows the hydrogen pellet dispersing near the outside of the plasma torus when it’s injected from outside the ring, where the magnetic field is weaker.
Samtaney thinks something more is going on. He believes the pellet ablates quickly at first, but the gas cloud shields it and slows ablation. As the gas becomes ionized and the ablated cloud moves away, “the pellet is exposed again and starts ablating faster” until another gas cloud forms and ablation slows again, Samtaney adds.
“It’s a fluctuation thing, and I think also the reason the fuel is pushed toward the core,” Samtaney says. He hopes his next simulation will give clues to whether he’s right.
Future research also will focus on simulating the pellet with greater resolution.
Samtaney also is working with Roman Samulyak, a researcher at the Department of Energy’s Brookhaven National Laboratory, and Paul Parks of General Atomics, a private research company.
Samulyak and Parks developed a detailed simulation of the area immediately around the pellet. The researchers want to merge this highly localized simulation with Samtaney’s global model.
“They have important parts of physics that are missing in my calculations, and I have important parts of physics that are missing in their calculations,” Samtaney says.